SAE 1008 CARBON STEEL
The AISI carbon steel 1008 has a manganese content of around 1.2% and a silicon content of approximately 0.4%. Chromium, molybdenum, aluminum, nickel, and copper are also found in carbon steels, albeit in much smaller quantities.
It offers outstanding formability and weldability, which encompasses spot and fusion welding, brazeability, and projection, among other capabilities.
Carbon steel with the AISI 1008 designation is often put to use in cold upset, cold headed, extruded, and cold pressed components and form. AISI 1008 in the cold rolled state is most often used for components that are either exposed or unexposed and may include modest amounts of welding, drawing, bending, or shaping.
Carbon steels are a kind of steel that have a high percentage of carbon as the primary alloying ingredient. These have a manganese content of around 1.2% mg and a silicon content of about 0.4%. (si). Carbon steels also include trace amounts of nickel, aluminum, chromium, copper, and molybdenum. These elements are present in very low concentrations.
UNS number is G10080 . Both the SAE and AISI numbering systems use the name sae 1008 steel grades for this material. Using resistance welding methods, AISI 1008 may often be welded without the need for any additional safety measures. micro metals manufactures SAE 1008 round bar as well as SAE UNS G10080 carbon steel castings using 1008 material. It has a somewhat poor electrical conductivity and, depending on the conditions, a moderately low tensile strength as well.
The characteristics of SAE 1008 steel include two typical varieties: cold-drawn 1008 carbon steel and hot-rolled 1008 carbon steel. Both of these variations are carbon steel. Applications for AISI 1008 steel include those dealing with furniture, household appliances, and automobiles.
1008 Steel Chemical Composition
Element | Content (%) |
Manganese, Mn | 0.30-0.50 % |
Iron, Fe | 99.31-99.7 % |
Sulfur, S | 0.050 % |
Carbon, C | 0.10 % |
Phosphorous, P | 0.040 % |
Carbon Steel 1008 Mechanical Properties
Properties | Metric | Imperial |
Tensile strength | 340 MPa | 49300 psi |
Density (composition 0.06% C, 0.38% Mn, 0.01% Si, annealed at 925°C) | 7.872 g/cm3 | 0.2844 lb/in³ |
Elastic modulus | 190-210 Gpa | 27557-30458 ksi |
Yield strength (depending on temper) | 285 MPa | 41300 psi |
Shear modulus (typical for steel) | 80.0 GPa | 11600 ksi |
Bulk modulus (typical for steel) | 200 GPa | 29000 ksi |
Elongation at break (in 50 mm) | 20% | 20% |
Poisson’s ratio | 0.27-0.30 | 0.27-0.30 |
Reduction of area | 45% | 45% |
Hardness, Brinell | 95 | 95 |
Hardness, Knoop (converted from Brinell hardness) | 113 | 113 |
Hardness, Rockwell B (converted from Brinell hardness) | 55 | 55 |
Hardness, Vickers (converted from Brinell hardness) | 98 | 98 |
Machinability (based on AISI 1212 steel as 100 machinability) The machinability of group I bar, rod, and wire products can be improved by cold drawing) | 55 | 55 |
THE THERMAL PROPERTIES OF AISI 1008 CARBON STEEL
Properties | Metric | Imperial |
---|---|---|
Thermal expansion co-efficient (@0.000-100°C/32-212°F) | 12.6 µm/m°C | 7 µin/in°F |
Thermal conductivity (composition of 0.06% C, 0.4% Mn; 0°C ) | 65.2 W/mK | 452 BTU in/hr.ft².°F |
Nanocrystallization of the surface of SAE 1008 steel with the use of single and multipass thread rolling processes
The layers that are formed on the surfaces of the single-pass and multi-pass thread rolled screws are the focus of this research, which investigates those layers. It has been noticed that as the number of passes increases, the deformation becomes more uniform, and the failure of thread laps does not occur on threads that have undergone three passes. On the surfaces of the thread rolled SAE 1008 steel, there is found to be a nanocrystallized layer.
The thicknesses and sizes of this layer are dictated by the degree of deformation. While the top of this layer contains grains of finer sizes, depending on the degree of deformation that occurred,
The grains became course and were orientated in the direction of the deformation. In the sample that was rolled with a single pass, the grains that were seen to be finer had a size of 136 nm when measured 15 millimeters inward from the surface of the root of the thread.
When compared to the bulk material, the surfaces of the all thread rolled samples have been seen to exhibit a considerable increase in their hardness. The number of passes has a very little impact on the difficulty; as the number of passes grows, the level of difficulty also rises marginally. On the other hand, layer thicknesses are increased so as to raise the pass number.
1 The first thing to note
The usage of threaded fasteners, which are considered to be one of the basic machine parts, as well as shafts that have an external thread is widespread across all areas of industry, particularly in the areas of mechanical applications and civil constructions [1].
As a result, the effectiveness of the procedures used in the manufacturing and design of threaded machine parts is likewise of utmost significance [2].
Machining and thread rolling are two procedures that are used to make the screws that are used in bolts and externally threaded shafts [3]. There is another text that goes into further detail on the process of thread rolling.
Because there is no chip formation in the bolt production process when using this method, the factors (microcracks, micro scratches) that shorten the usage life of bolts are prevented.
These factors include the formed notch effect at the bolt surface via the cutting tools. As a result, the bolts can be obtained with a high surface quality [5, 6].
Studies on the thread rolling process typically focused on the effects on the mechanical properties [5, 7-9] and the finite element modeling of the thread rolling process [5, 7-17].
Despite the fact that the thread rolling process has been widely used as a production method for a considerable amount of time, these studies were conducted. On the other hand, there hasn’t been a lot of study done on the microstructures of the threads or how the thread laps arise during the single or multipass thread rolling processes.
None of these investigations, however, have been able to detect and identify the surface layer that contains nano-scale grit [7, 13].
As a consequence, the nano-sized microstructure that was created at the surface of the material as a result of the single and multi-pass thread rolling process was researched and described in depth during the course of this particular study.
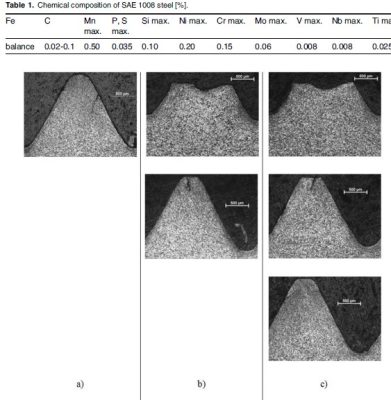
2 Experimental study
The inquiry required that the threaded fastener be fabricated using SAE 1008 carbon steel as the parent material. This was done in order to facilitate the findings of the research.
The constituent chemicals of the parent material are laid out for your inspection in Table 1. After the creation of samples with dimensions of 18 millimeters by 300 millimeters, a production run of an M20 screw was carried out with the aid of a two-die cylindrical thread rolling machine (FK20 Gokceoglu). For the process of thread rolling, die sets constructed of alloy steel measuring 250 millimeters were used.
This method of rolling thread was executed at room temperature and without the use of any kind of lubricant. The rotation speed of the dies is maintained at a constant 45 revolutions per minute throughout the whole of the thread rolling processes.
Before the production of threads, they were rolled in single-pass, two-pass, and three-pass multi-pass configurations. This was done so that the microstructural and mechanical changes that occur on the surface of the thread could be investigated.
The screws had to have their cross-sections cut in order to carry out the metallographic examination and the hardness testing. After that, the samples were physically polished using sandpapers of various grit sizes. Finally, the samples were brought to a mirror finish using diamond paste down to 1 mm so that the layer morphology could be identified. After the mirroring was complete, the samples were allowed to etch in 3% Nital at room temperature while the solution was being agitated.
In order to determine the surface properties of the screws, microstructural studies as well as a test of the screws’ hardness were used.
In order to carry out microstructural research, we used an optical microscope (Nikon, Epiphot 200), a scanning electron microscope (Jeol 6060), and a technique of atomic force microscopy (Nanomagnetics, ezAFM) with a scanned area of 5.35 mm2.
The DUH-W201S is a microhardness tester that was manufactured by Shimadzu. It was used to determine the degrees of hardness of the materials. In the course of the research that was carried out, a Vickers indentation was used, and the amount of force that was used was 25 mN. The Microvickers hardness scale was traversed using a force that lasted for ten seconds and had a weight of fifty grams.
Hardness traverses were carried out all the way through the thread, starting at the root and finishing in the middle of the thread.
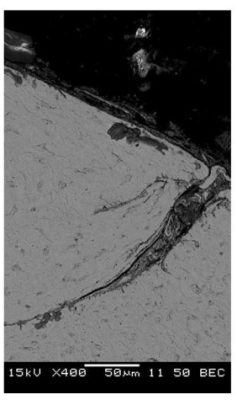
The results as well as the conversations
3.1 Cross-sectional microscopic structure of the thread.
The inability of the thread lap to develop at the surface of the screw during the single pass of thread rolling may be seen in cross-sections of thread-rolled screws, as shown in Figures 1 and 2.
One of the most prevalent and undesirable faults in the process of thread rolling is the formation of thread laps [18]. These failures are the result of design flaws that produce heterogeneous deformation, such as the misalignment of the thread roll dies, the quality of the blank, poor die design, tilting of the blank, slippage of the blanks, and the condition of the rolling machine [19, 20].
Thread laps failure did not arise on the 3-pass threads, as shown in Figure 1b and c. This is because the plastic deformation becomes more uniform as the number of passes in the thread rolling process increases.
When compared with the grains of the parent material, the grains that developed on top of the thread surface were finer as a result of the significant levels of cold deformation that occurred.
These granules have a size on the nanoscale and have developed on the surface of the threads. On the surface of each treated sample, this nano-crystallized layer was able to develop.
Figure 3 demonstrates quite clearly that it is a layered structure that has grown on the surface as a result of the orientation. The processing of grain became more obvious, particularly near the base of the threads. Figure 3 shows that the grain boundaries in this layer cannot be observed clearly using an optical microscope. Figure 3 illustrates how the form and dimensions of grains vary as one moves from the surface to the interior areas of the material.
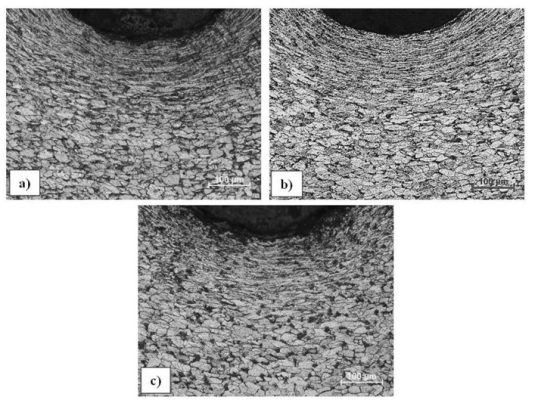
The grain morphology in the nanocrystallization layer features structures that are elongated and equiaxed, as shown in figure 4, which was obtained using atomic force microscopy on pictures of the nanocrystallization layers. Figure 4a shows that the granules at the very surface are equiaxed and exceedingly fine.
Figure 4d shows that the grains that lie underneath the equiaxed grains are aligned in a direction that is perpendicular to the path that the plastic deformation is taking.
The quantity of distortion causes the grain sizes to get increasingly smaller as they approach the thread surface. In every sample, the surface of the thread roots was the location where the formation of the smallest grains occurred.
At a distance of 15 millimeters inward from the surface of the thread root, the average grain size decreased from 20 millimeters to 136 nm, 214 nm, and 332 nm, respectively, after a single pass, two passes, and three passes, respectively.
Because of the significant degree of surface deformation, the precipitates were spread evenly throughout the top of the surface (Figure 4a, b). Ever since the formation of precipitates at the grain boundaries between 60 and 120 millimeters away from the surface, as seen in Figure 4c and d.
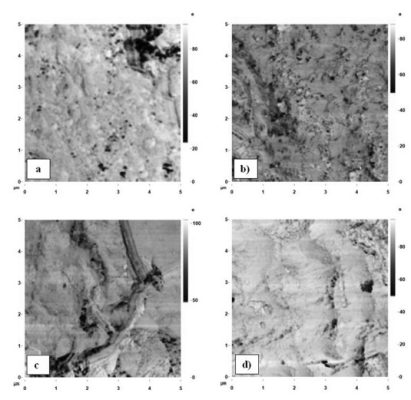
As the amount of deformation that occurs every pass is increased, there is a modest reduction in the grain sizes of the surface area. Because the amount of distortion that occurs during each pass is reduced throughout the process of multipass thread rolling. Grain size at the surface decreased in comparison to multipass samples as a direct consequence of the large amount of deformation that occurred during the single pass. This phenomenon is also seen in techniques involving extreme plastic deformation [21].
On the other hand, micro and nano-sized fractures and holes appeared, particularly on the surfaces of the single pass threads (Figures 5 and 6). Dislocation motion may also be observed quite well in the three-dimensional pictures obtained from atomic force microscopy (Figure 5).
At the surface of the threads, the thickness of the nanocrystallization layer does not have a uniform distribution. The thicknesses of this layer are greater near the roots, and they continue to get thicker as the amount of deformation that is applied rises. One hundred, one hundred fifty, and one sixty millimeters are the relative thicknesses of the nanocrystallization layer at the root for single, two, and three pass threads.
Below the nanocrystallization layer, there was also the formation of a zone that includes grains that are aligned. Figure 3 shows that these grains are aligned in a direction that is perpendicular to the direction of the plastic deformation.
3.2 Hardness behavior
Following the thread rolling procedures, a substantial improvement in surface hardness was seen across all of the samples when compared with the parent material (Figure 7). While the parent material has a hardness of just 200 HV, all of the sample surfaces had hardness values that are more than the threshold of 250 HV0.05. As one moves from the surface to the inside of the material, the hardness levels drop. In every sample, the levels of hardness that were measured at the thread roots were found to be the greatest (Table 2). These findings are consistent with the research that has been published before [22].
It should come as no surprise that the work hardening caused by the thread rolling process led to an increase in the hardness values after each pass. This increase was proportional to the number of passes. However, these high hardness values at the surface are not simply the product of work hardening but also grain refining, which is known from the Hall-Patch relation [23].
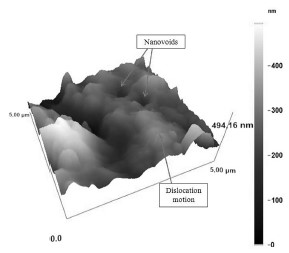
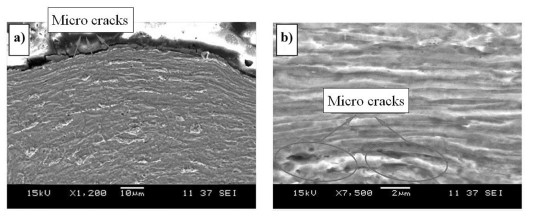
The surface of the three-passed thread has the greatest hardness rating of any other part of the thread. This figure for the hardness is quite close to the surface hardness of both single and two pass threads.
4 Concluding Remarks
As the number of passes increases, the homogeneity of the plastic deformation increases as well. As a consequence of the homogenous deformation, thread lap failure reduced in the sample that was subjected to two passes but did not take place in the sample that was subjected to three passes. Because of the significant degree of deformation, a nano-crystallized layer has developed on the surface of each of the samples. Images obtained by atomic force microscopy reveal that the grain morphology in the nanocrystallization layer contains features that are both elongated and equiaxed.
As the degree of deformation in the material increases, the grain sizes will continue to decrease from the inside to the outside of the material. In every sample, the surface of the roots was the location where the formation of the smallest grains occurred.
At the surface of the root of the single-passed screw, the lowest grain sizes (136 nm) were measured.
On the other hand, fractures and holes of microscopic and nanoscale dimensions were seen at the surface areas of the threads that were formed by a single pass of the thread rolling procedure. Below the nanocrystallization layer, there was also the formation of a zone that includes grains that are aligned.
These grains are aligned in a direction that is orthogonal to the path that the plastic deformation is taking. When compared with the original material, the surface hardness of each sample significantly increased after being treated with the compound. As one moves from the surface to the inside of the material, the levels of hardness drop
The three-pass thread yielded the greatest possible result for the hardness dimension. On the other hand, the difference in toughness is not very noteworthy.
Soruce : https://www.researchgate.net/publication/263851775_Mechanical_properties_of_friction_stir_butt-welded_Al-5086_H32_plate